ATLAS Experiment
The ATLAS (A Toroidal LHC ApparatuS) detector is a particle physics experiment at the Large Hadron Collider (LHC) at CERN (Conseil Européen pour la Recherche Nucléaire). The LHC is the most powerful particle accelerator built to date. This hadron machine is extending the frontiers of particle physics due to its unprecedentedly high energy. It is designed to collide proton beams 40 million times per second at a centre-of-mass energy of 14 TeV. Since the start of the LHC operation in 2009, the accelerator has been improving its performance: increasing the luminosity and doubling the beam energy, until reaching 13 TeV in p-p collisions.
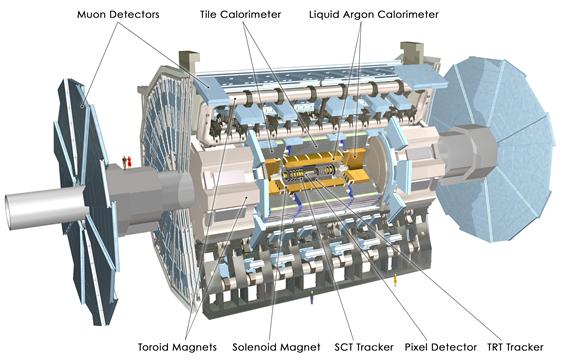
It is a general purpose detector built to fully exploit the physics produced by the LHC, from precision physics within the Standard Model all the way to new physics phenomena beyond it. Thanks to the good operation of the machine and detectors in the first data-taking run, some of the major goals of the ATLAS detector, such as the discovery of the Higgs boson in 2012, have already been achieved. In the second data-taking run, ATLAS achieved an analysis milestone by releasing 100 results using full Run-2 dataset. One of the major results was the evidence of the extremely rare four top-quarks production. ATLAS is all set to start the third data-taking run in 2022.
At the LHC design luminosity, a large number of particles emerge from the interaction point every collision creating a high charged-track multiplicity in the detector. In order to operate under these conditions, the ATLAS detector is equipped with fast and radiation-hard electronics and sensors. In addition, the high granularity of the detector allows handling the huge particle flux and reducing the influence of overlapping events.
The ATLAS detector is nominally symmetric with respect to the interaction point. This detector weights 7000 tons and it is 45 m long and 22 m tall. Its large size together with a powerful magnet system allow for a good momentum resolution of the charged particles. The magnet system is composed of a thin superconducting solenoid in the inner part and three large superconducting toroids in the outer region of the detector.
- Inner Detector(ID): It is immersed in a 2 T solenoidal field and is responsible for the pattern recognition, the momentum measurement of the charged particles and the reconstruction of the primary and secondary vertices. This is achieved using a combination of discrete, highly granular semiconductor pixel and strip detectors in the inner part of the tracking volume, surrounded by a straw-tube gaseous detector (TRT) with the capability to discriminate electrons from charged pions.
- The ID is then followed by the calorimeters, which are the responsible for measuring the energy of the particles. ATLAS uses a high granularity liquid-argon (LAr) electromagnetic sampling calorimeter, with excellent performance in terms of energy and position resolution. The hadronic calorimetry consists of iron and scintillator-tiles in the central part of the detector, and LAr technology in the forward region, matching the outer limits of electromagnetic calorimeter.
- The outermost detector is the Muon Spectrometer (MS) which includes three large superconducting air-core toroids, generating a strong bending power in a large volume within a light and open structure. An excellent muon momentum resolution is achieved by means of three layers of high precision tracking chambers. The good timing resolution of the muon detector also plays a crucial role in the trigger system.
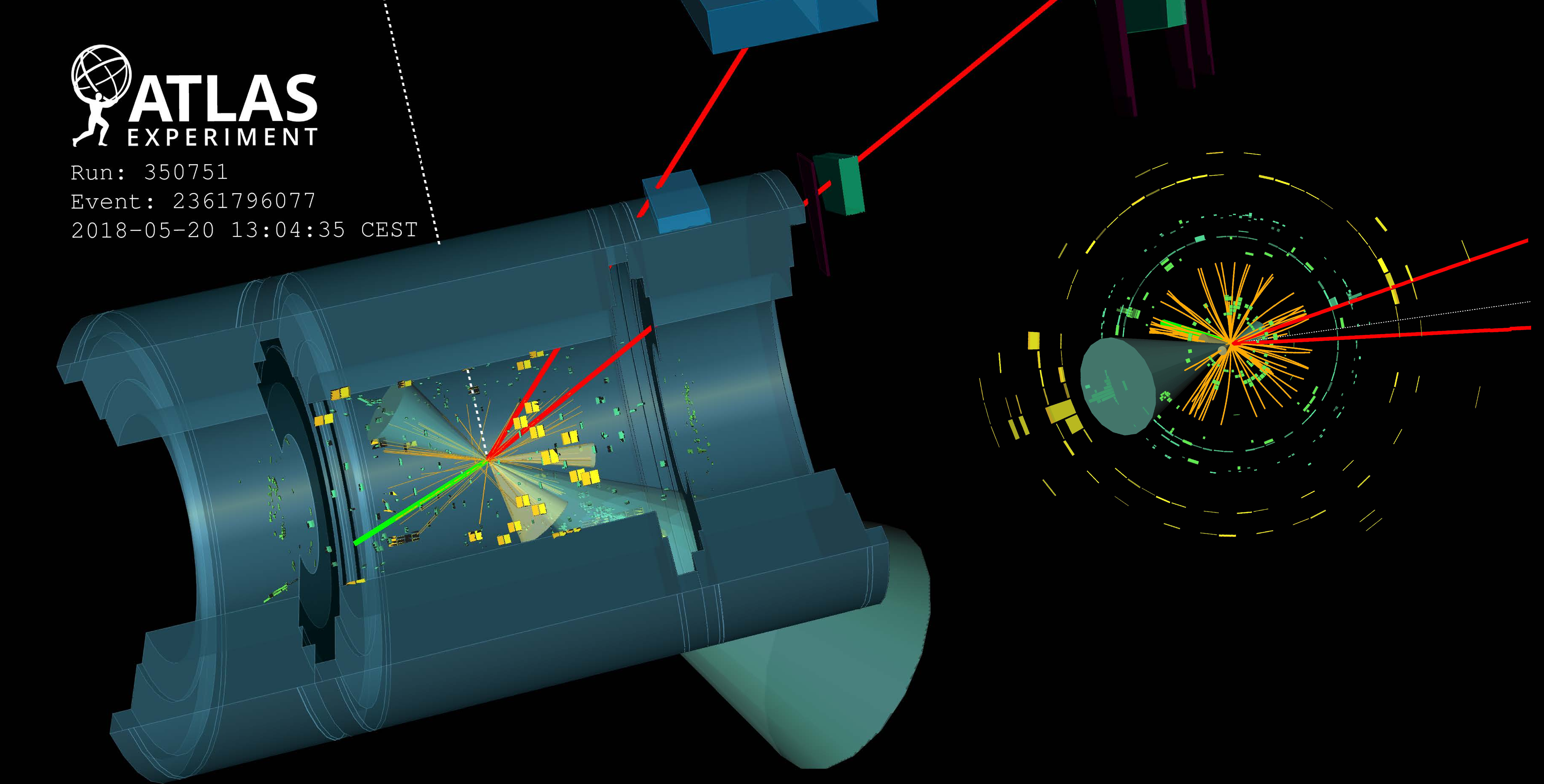
The top quark was discovered in 1995 by the Tevatron experiments CDF and D0. Its discovery was a great success of the Standard Model (SM) because it confirmed the existence of the predicted weak isospin partner of the bottom quark. At hadron colliders, the top quark is predominantly produced via the strong interaction and it decays in a short time (∼10−25 s) before hadronising. Its decay is almost exclusively through the single mode t → Wb (> 99%).
There are many motivations to study the top-quark physics at the LHC. Among others, the top quark is the heaviest fundamental particle (~40 times larger than the following massive quark) and its mass is close to the electroweak symmetry breaking scale. Therefore, a precise determination of its mass allows stringent tests on the SM. In addition many beyond the SM heavier particles decay into top quarks. Hence studying in detail the properties of the top quark, can provide handles on new physics.
At the LHC, millions of top-quark pairs are produced as a result of the proton-proton collisions. These events provided, at the beginning, an important calibration tool for ATLAS, playing a key role in the detector commissioning phase. Beyond that, the huge amount of collected data has allowed precise measurements to be performed, for example the top-quark mass, and also to measure the top-quark cross sections for a broad range of channels and topologies.
Active research
- The determination of single top-quark cross-sections is one of the measurements that are being carried out in our group. The cross-sections are an important test of perturbative QCD at high transverse momentum and a deviation of the expected SM cross-section may be a hint of BSM processes. The single top quark is produced via the electroweak interaction through three different processes, so-called, s-channel, t-channel and Wt production. The group is mostly involved in the Wt and t-channel cross-section measurements.
- Our group is also involved in measuring the coupling between a top quark and the Higgs boson with the data from LHC Run 2. If the Higgs boson coupling is exactly as predicted in the Standard Model, the production of a Higgs boson in association with a single top quark is expected to be very small. However, non-standard couplings could enhance the cross-section significantly. As a first step towards a measurement of tH production, tZ production is also been studied by our group.
- In addition, a search of vector-like quarks (VLQs) with events containing a top quark and a W boson in the final state is ongoing. VLQs appear in several extensions of the SM, such as extra dimensions, super-symmetry and composite Higgs models, in which they solve the SM hierarchy problem.
In the past out group was also involved in searching of new processes. As mentioned above, in the SM the top quark decays almost exclusively to a W boson and a b quark. The decay of the top quark to other quark flavours mediated by neutral bosons, so- called flavour changing neutral current (FCNC), is forbidden at tree level and highly suppressed at higher orders. However, some extensions of the SM increase the FCNC production rates and therefore the anomalous single top quark production (qg ➝ t) was studied.
STYX stands for Straw Tube Young student eXperiment. The main aim is to offer students an opportunity to study tracking of cosmic particles using state-of-the-art detectors. The big advantage is the vastly reduced complexity compared to modern large scale experiments at the LHC while retaining the basic problems and questions of charged particle tracking. STYX is a joint project of two groups by Prof. Brock and Prof. Desch and is using modules which were formerly part of the ZEUS Straw Tube Tracker (STT) to detect secondary cosmic muons. A setup with two or more of these modules allow not only a position measurement but also the reconstruction of angular distributions of the tracks. These modules are flooded by ArCO2 which is provided by a supporting gas system. Two scintillators, above and below the modules, with photomultipliers are used as a trigger system. Collected data is then processed using a self-developed software package in order to reconstruct track objects and visualize them for a better understanding.
The STYX experiment measures drift times in gas detectors to reconstruct charged particle tracks from cosmic muons. The main aim of the experiment is to provide a not too complex setup where students get an idea of the different aspects of a typical high energy physics experiment. STYX is employed in the master student's lab course and more detailed studies are done in bachelor or in master theses programs. The topics covered by STYX are:
- Theoretical basic knowledge of cosmic radiation
- Elementary electronic setups
- Understanding of electronic components
- Scintilator detectors as trigger systems
- Drift time measurment and determination of drift velocity in a gas detector
- Data taking system and reconstruction algorithms
- Working with a modern software package to analyse large datasets
- Monte Carlo simulation and comparison with cosmic data
- Different combinations of these questions or a more detailed look at single topics are the possible tasks for the students.
ZEUS was one of two colliding-beam detectors at the particle accelerator HERA at the Deutschen Elektronen Synchrotron, DESY, in Hamburg. It was the only ep collider in the world and was built in the 1980s. Positrons or electrons and protons were collided between 1992 and 2007. After 8 years of successful data taking the accelerator as well as the detectors underwent a major upgrade. HERA achieved a factor of 4 higher luminosity and additional lepton beam spin rotators in the neighborhood of the two general purpose detectors enabled physics studies with longitudinally polarised beams. The ZEUS detector was also extended at this time by several additional components: Micro-Vertex-Detector MVD, Straw-Tube-Tracker STT, a spectrometer for small-angle scattering (6m-Tagger), and a new luminosity detector. During the last three months of data taking in 2007 the proton beam energy was varied in order to measure of the longitudinal structure function FL of the proton.
The general task of the ZEUS collaboration was to investigate the interactions between electrons/positrons as probes on the one hand and the constituents of the proton (quarks and gluons) on the other. The aim of the ZEUS research program was to increase the current knowledge about basic mechanisms of the strong and electroweak interactions in the Standard Model as well as to discover new phenomena beyond the Standard Model.
The group in Bonn was involved in several detector components both from first and second development phases. Additionally we analysed ZEUS data and investigated several problems of particle physics. You can find more details about the detector on the ZEUS web page .
Analyses mostly dealt with different questions from the "Heavy Flavour" physics group; especially using leptons from semileptonic heavy quark decays. Besides this several theses considered the topic of meson production, e.g. to extend the kinematic region by fully utilising the power of the STT. Other analyses covered the field of inclusive neutral current and charged current cross-sections as well as measurements of diffractive processes.Another important part of our activities concentrated on the STT included the software development for data readout and processing. Several software packages have been written and extended for the data quality monitoring, the processing and reconstruction up to the analysis level and the event visualisation. To guarantee stable and high data quality the visualisation and monitoring in "data quality monitoring (DQM) plots is of big importance. Various theses about the track correction and reconstruction made it possible to integrate the forward detector into the combined tracking system of ZEUS. A lot of effort was spent on the data correction and Monte Carlo simulation to describe the specific energy loss, dE/dx, which was measured by the Central Tracking Device, CTD. Another example of our software activities is ZeVis, a tool for event visualisation of both recorded as well as simulated events.
The ZEUS Bonn Group has been involved in the work on various detector components of both the first as well as the second phase of the HERA running. One example is the construction of a testing facility for the calorimeter read-out electronics and building a prototype of the Transition Radiation Detector, TRD. Beyond the construction several theses have been devoted to testing and deployment of the detector.
The group in Bonn has played a significant role in the planning, development and support of the Straw Tube Tracker, STT, which was a part of the sophisticated forward tracking system. The work included studies on the track reconstruction, simulation and efficiency calculation, calibration, the control of the data quality and other tasks. The right picture shows the compound and cabled Forward Detector, FDET(FTD3/STT2/FTD2/STT1/FTD1). Elements of the STT are now included in a advanced lab. course experiment, STYX, which is supported and further developed by the group.
Latest results and thesis opportunities
Publications
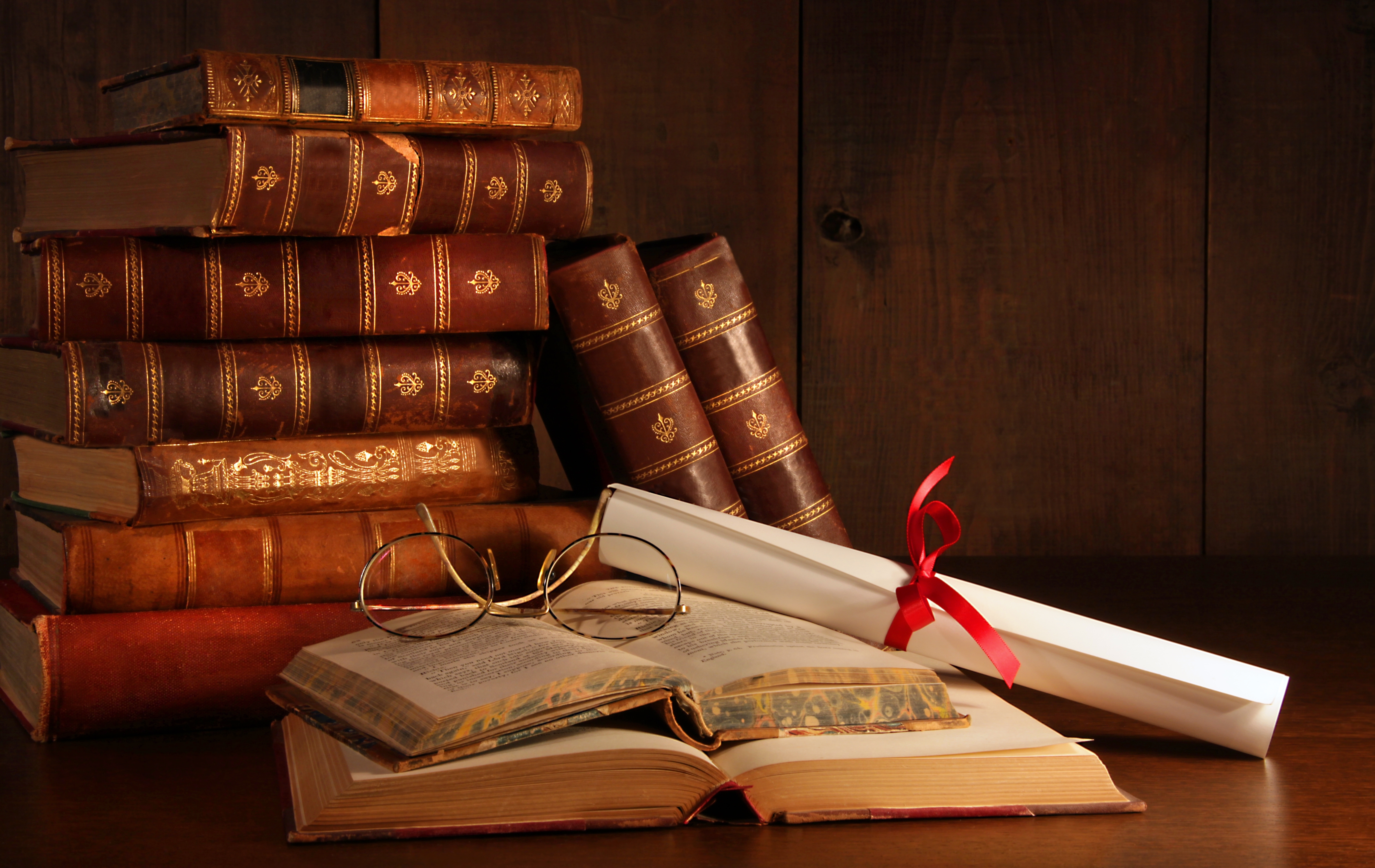
Here you can find all the publications by Brock group members and alumni.
Open positions
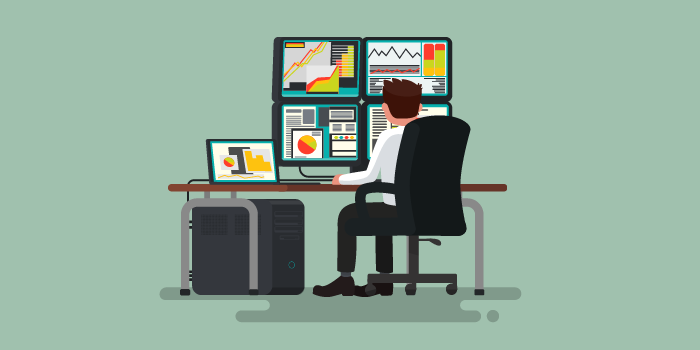
Here you can find informaion about possible bachelor and master thesis topics.